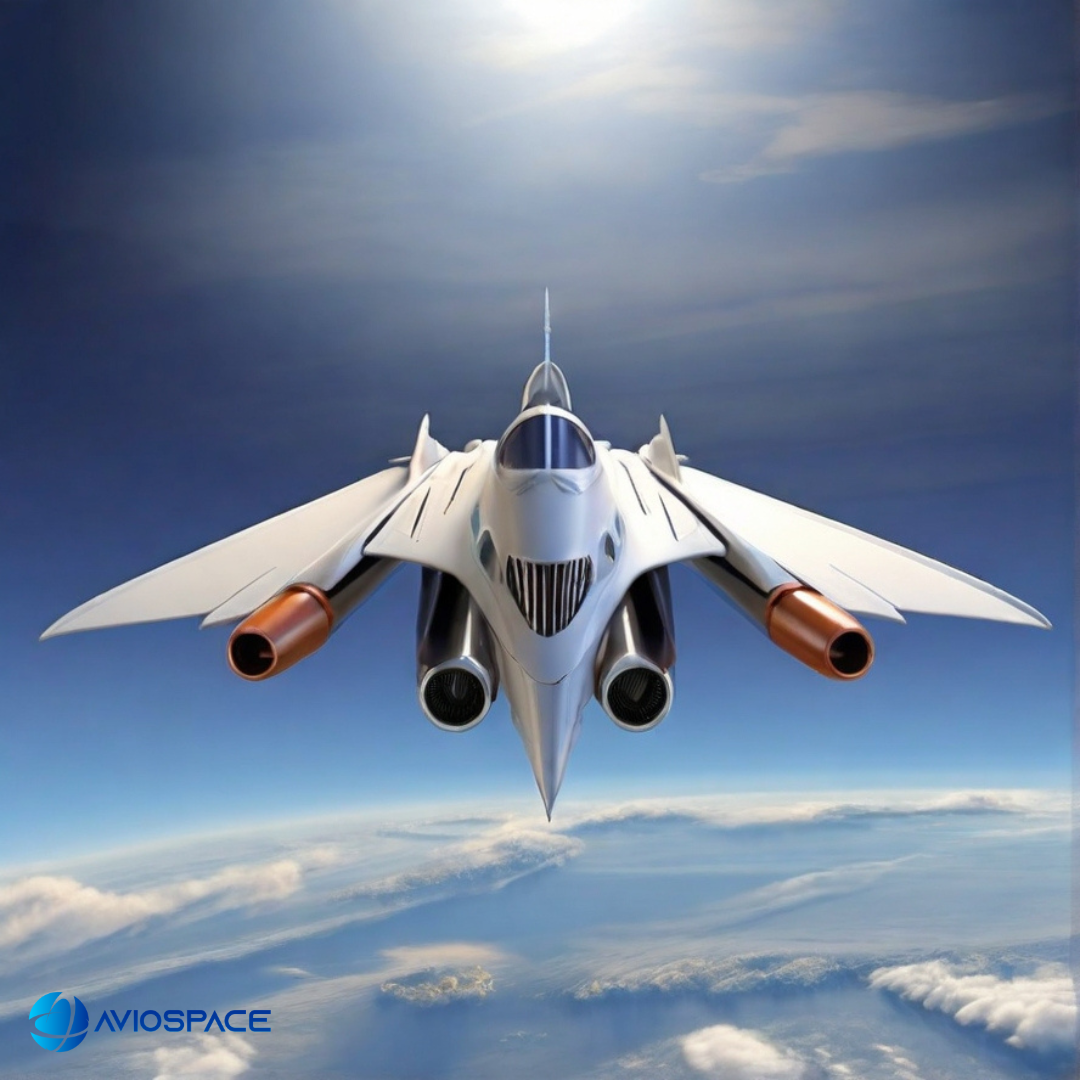
To triumph over the limitation of accessing space, any air-cum-space craft requires a complex mesh of systems that are not limited to propulsion, thermal protection, life support, navigation, and control. As opposed to aircraft using atmospheric air as oxidizers and only having to carry fuel, spacecraft need to carry their own oxidizers (in addition to fuel) that are essential for combustion throughout the flight time. Outer space consists of inadequate atmospheric(Earth-like) components where little or no air may be present. This prevents any aircraft from flying in vacuum-mimicking conditions.
If any aircraft had to fly to space, it would also have material-related restrictions. The heat shield on a spacecraft’s surface (that absorbs and dissipates heat) is required to sustain a temperature as high as 1600 Degrees C. The propulsion system is intricate and requires flawless activation of different fuel feed and ignition systems.
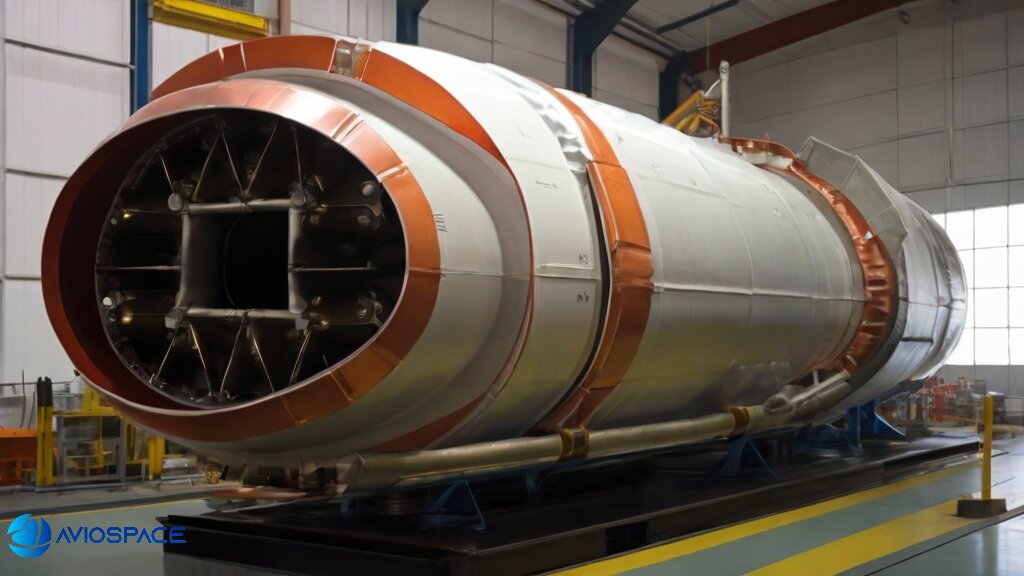
In any flight that inches upward to space, the space vehicle is equipped with life support systems that simulate the atmospheric environment for crew survival. The internal cabin pressure is usually standardized to 1 atmospheric pressure. Without these systems, spaceflight would be unviable to humans, not to mention the animals that are sent to space for experimentation.
Defying Earth’s Gravity
To escape the Earth’s atmosphere, an object has to attain an escape speed- a minimum velocity that any object requires if it is to break the barrier of Earth’s gravity and atmospheric drag. Calculations show that any object that achieves a speed of 11.2 km/sec can get out of the Earth’s atmosphere. Spacecraft in which objects such as the James Webb Telescope that are sent for deep space exploration only need to attain higher than this speed to escape the influences of the gravitational pull of the Earth.
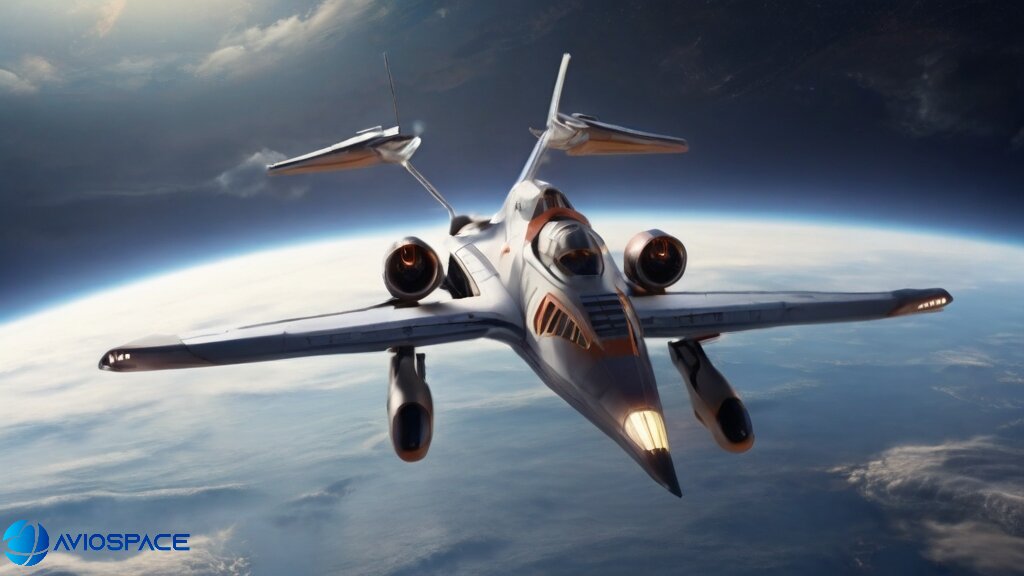
Supersonic Flight and the speed of sound
The speed of sound is usually expressed in terms of a dimensionless quantity, i.e. Mach number. Any object that is flying at the speed of sound has a Mach number of 1 or simply M1. Supersonic aircraft fly at a speed more than the speed of sound, with Mach numbers ranging above one and less than 5. As the aircraft reaches supersonic speed, all the airflow on the body transitions from subsonic to supersonic.
Any aircraft that operates at M>1 produces shock waves. In addition, compressibility effects have a significant play in the flow of gases. This piles up the pressure at the front of an aircraft and, thus, leads to higher drag production.
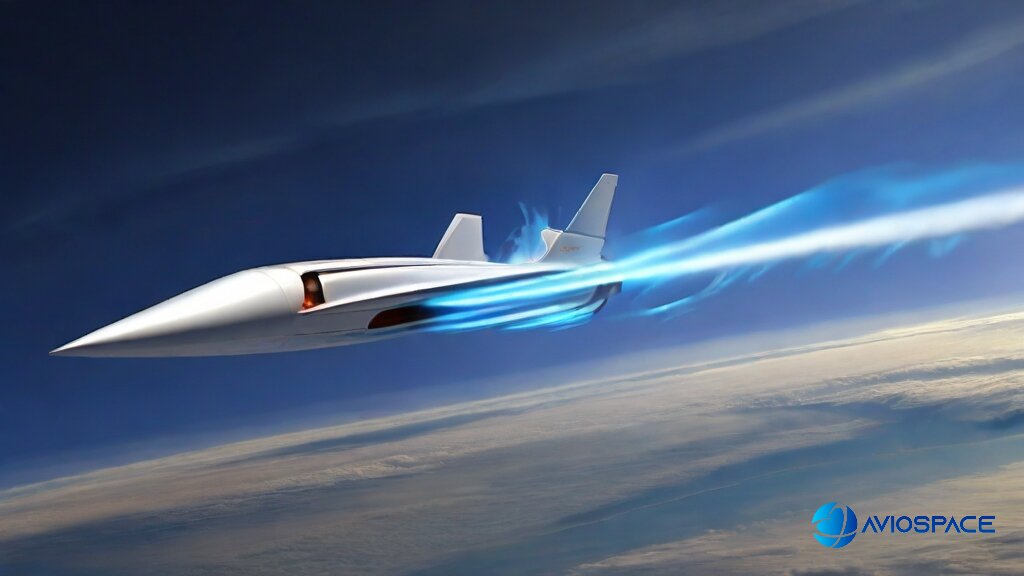
The Only Aircraft in Space
North American X-15, a rocket-powered hypersonic aircraft (aircraft flying at five times the speed of sound or above are known as “hypersonic”), reached an altitude as high as 354,200 feet- making it the aircraft that has attained the greatest vertical distance. As this aircraft mimicked rockets too much, there were serious considerations during the incipient days of Project Mercury about whether an extended version of the X-15 would be the first spacecraft to carry humans to space.
Definition of spaceflight
Spaceflight refers to the act of sending an object into space that is either accompanied by a human or without one. The space region starts with the passing of the Karman line. This imaginary line is 100 km above the Earth‘s surface where atmospheric air constituents are relatively less dense to sustain conventional aircraft flights.
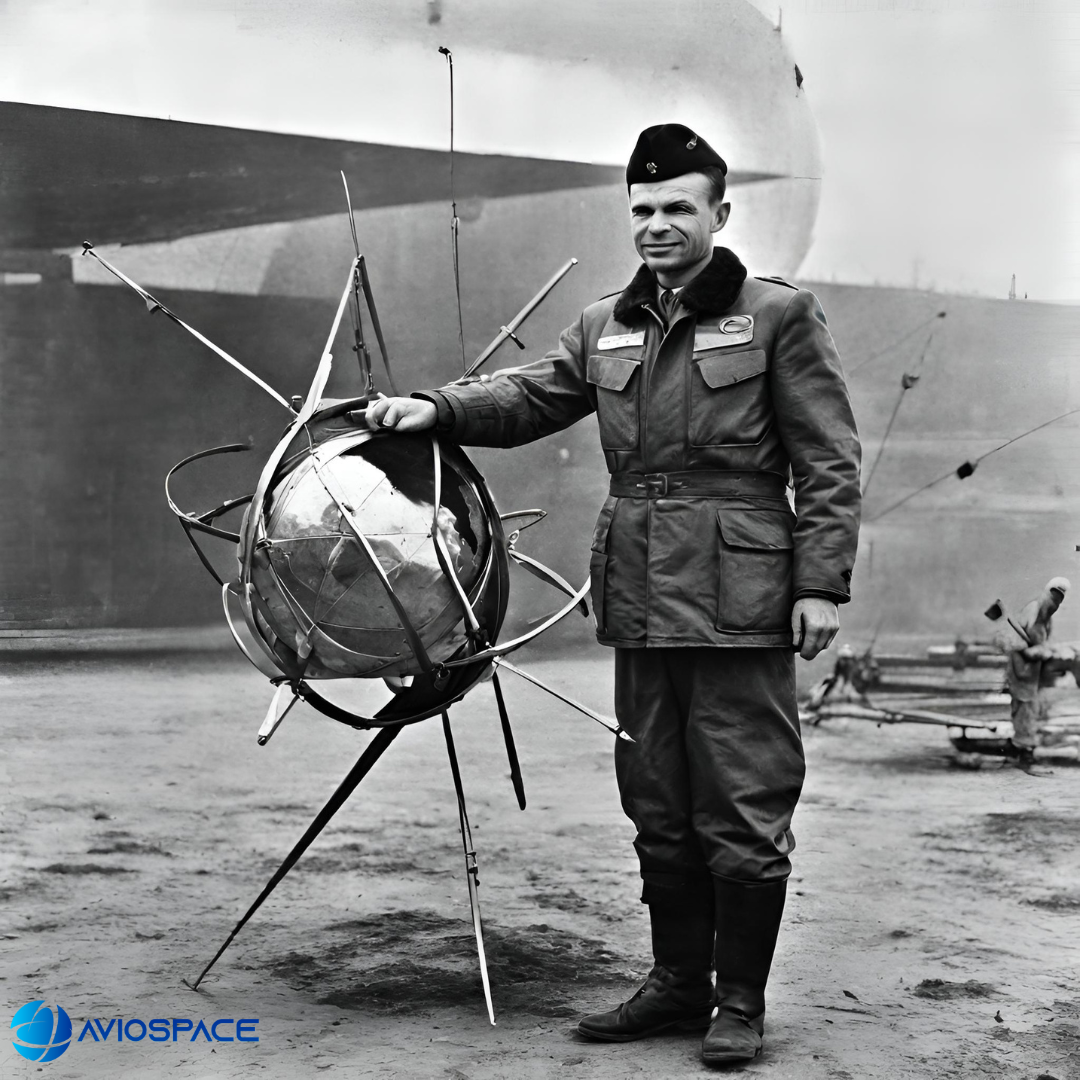
The past is rife with examples of massive failures to make spaceflight successful. This is because spaceflight necessitates a non-air-breathing engine to fly above the atmosphere. A scarcity of refractory materials that are resistant to high temperatures was another reason why spaceflight remained in the confines of mathematical calculations.
Brief History of Spaceflight
Humans started exploring the vastness of space with the successful attempt of the Soviet Union’s Sputnik into space in 1957. Yuri Gagarin’s first Flight into space on April 12, 1961, was an event that was of utmost significance for humans, as it opened up avenues of venture into space.
Apollo’s Flight in pioneering human landing on the Moon as well as the first Flight of reusable Space Shuttle spacecraft, broke barriers set by materials and engines. They did so by utilizing synthetic materials and thermal coating tiles which were engineered with precision and produced tons of thrust.
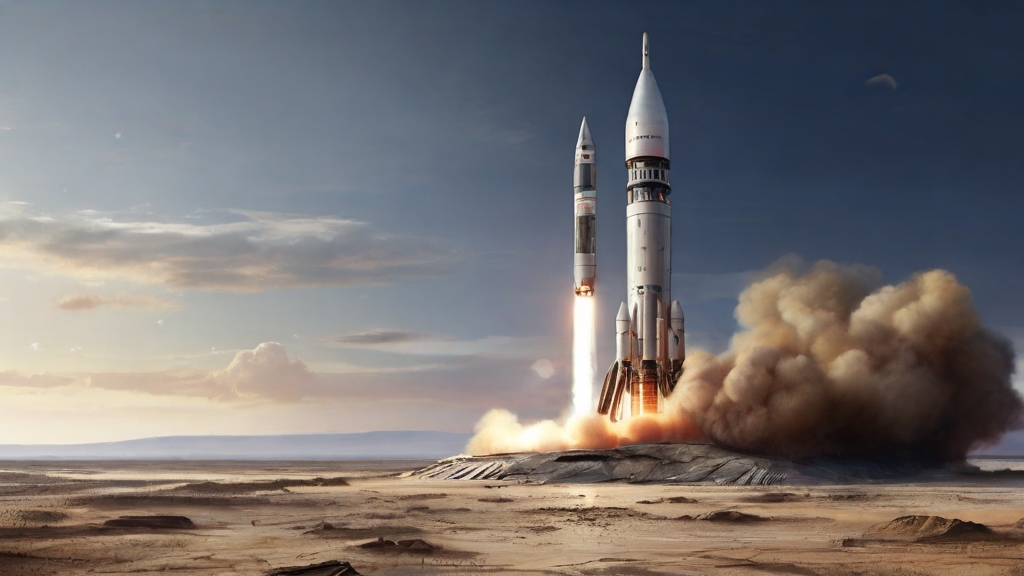
The milestone that was achieved using Apollo almost 50 years back is now going to be accomplished with the resurgence of NASA’s yet another crewed mission to the Moon, namely the Artemis Mission, producing almost 8.8 million pounds of thrust at liftoff (In comparison, Saturn V requires just 7.5 million pounds). Once this program becomes full-fledged, leveraging the Orion Spacecraft will allow us to travel as far as Mars and, in decades to come, further deep into space.
Even though there has been no record of any aircraft launched into space, the North American X-15 had frequently crossed the Karman line. But the fact that it was a cross between an aircraft and a rocket meant that we can’t quite call it an “aircraft that flew to space”.
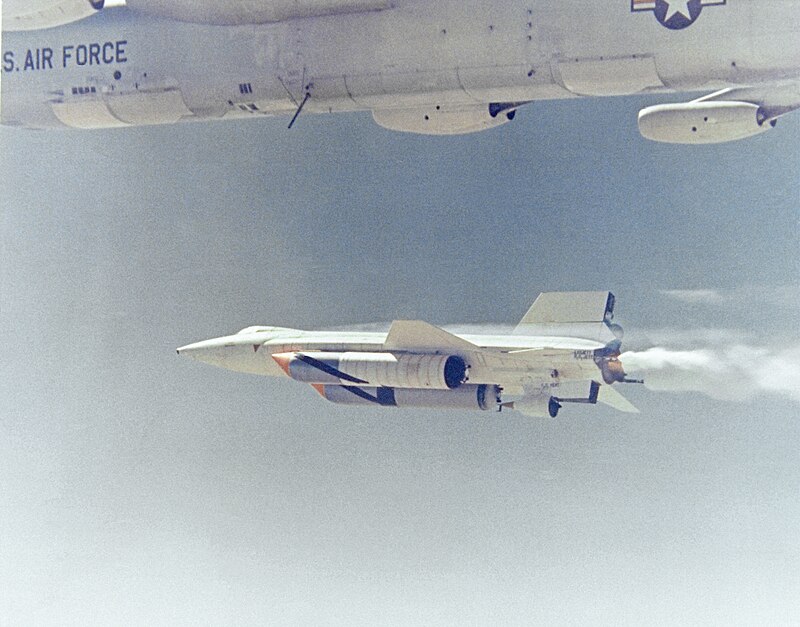
The North American X-15 is equipped with rocket ignition engines.
Understanding Limits of Supersonic Flight to Space
Structural Limit
Supersonic aircraft utilize a special variant of material that can withstand deterioration that can be caused by great speeds. This is because with higher speeds come higher thermal stress. Aluminum alloys can be utilized as a primary structural material, even when speeds are greater than M1.
The higher the speed is, the higher the thermal stress the aircraft experiences. As reaching space requires a staggering hypersonic speed (~M33), different parts are assembled to make it aerodynamically fit taking factors such as drag into consideration. As various structures experience drags and temperature fluctuations differently, the expansion(rates) of these structures is also quite different.
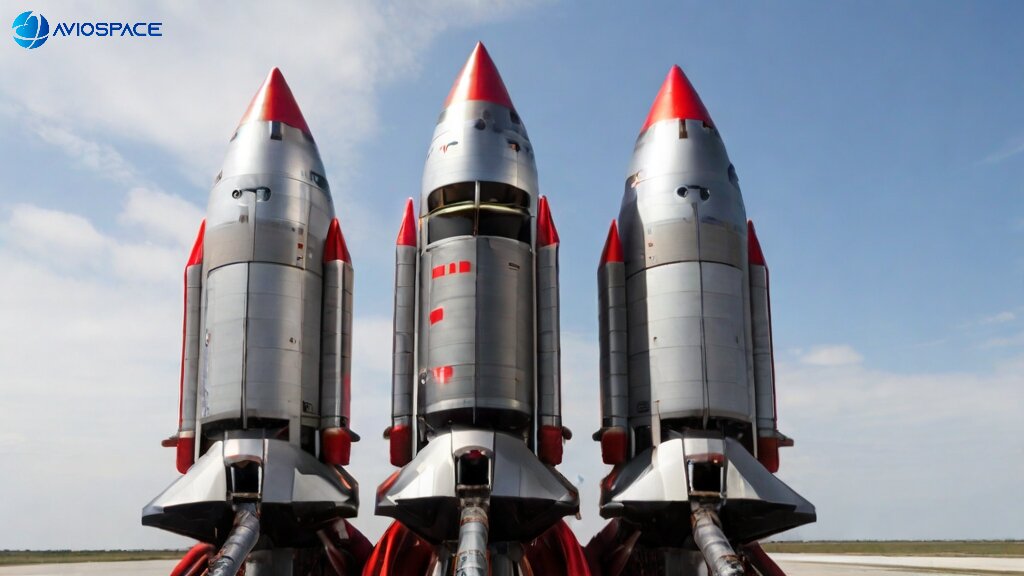
Thermal stresses at such staggering temperatures can also cause thermal fatigue and structural failure- two things that limit a supersonic spacecraft from flying to space.
Taking Temperature into consideration
The temperature that a spacecraft during its reentry to the Earth is subjected to ranges from 3000°C to several thousand. The base and front faces of the spacecraft have to bear the maximum brunt of the exorbitant temperatures. And without the installment of a thermal protection system, the aircraft has no chance to withstand such temperatures.
One of the fastest military aircraft, i.e. MiG 25 Foxbat, which is capable of flying above Mach number 2.8 though not completely resembling the characteristics of the Space Shuttle orbiter, is comparable to the Orbiter for its delta wing configuration and plane-like design.
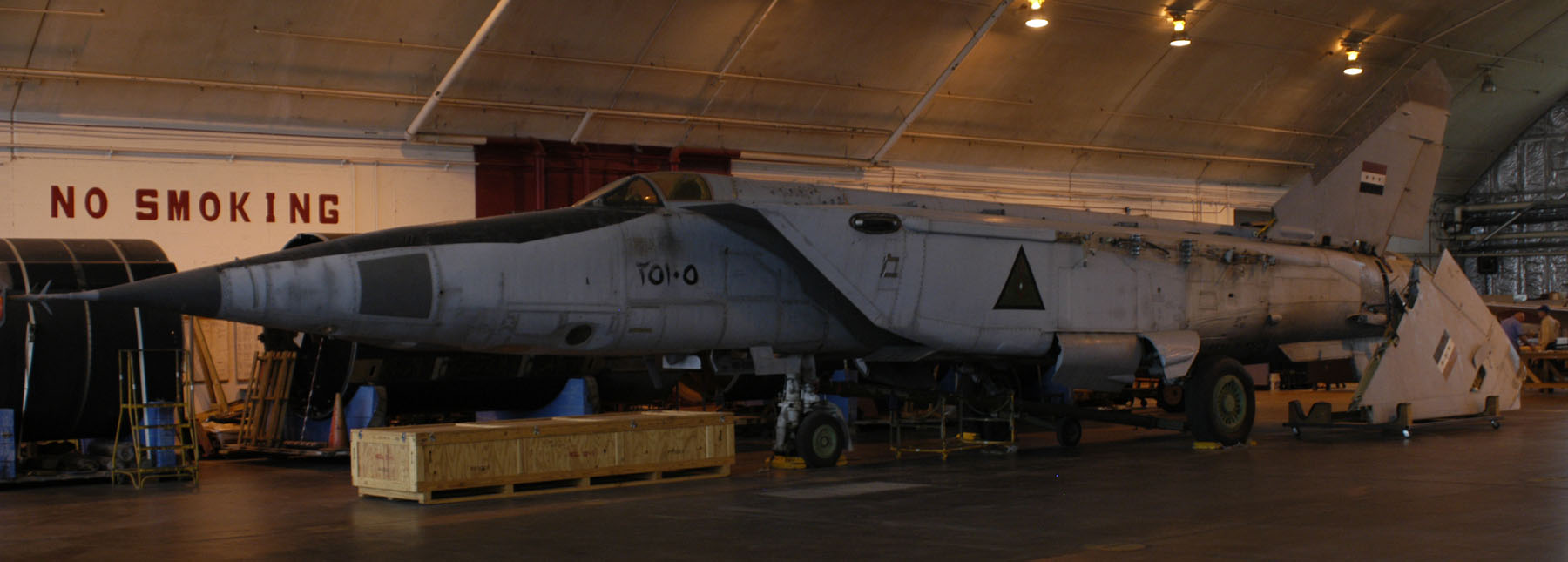
A Mig-25 is a fighter craft that has tinctures of the elements of the delta-wing configuration.
The fuselage of MiG 25 Foxbat was designed predominantly from a high-strength alloy called stainless steel. Aluminum alloys, well known for their light weight and strength, were used in wings and control surfaces.
Different types of material
The case for Titanium
Titanium was used in the critical areas of the aircraft which required high strength and low weight, in addition to having the ability to withstand high temperatures. Titanium was able to maintain its structural integrity and durability even when flying at a speed as high as Mach number as high as 2.8. But we can’t quite make an aircraft out of Titanium itself because of weight considerations. Though Titanium is lighter than steel, it isn’t as light as aluminum.
Aluminum and its usage
The primary structure of the Space Shuttle Orbiter was predominantly made up of Aluminum alloys. However, if the Orbiter were to travel almost 25 times the speed of sound, the material wouldn’t be able to stand the prolific thermal stresses resulting from atmospheric friction in the reentry phase. Therefore, different materials were employed in its structural design. Reinforced Carbon was used on the wing’s leading edges and nose cap to prevent damage from temperatures as high as 2,300°F.
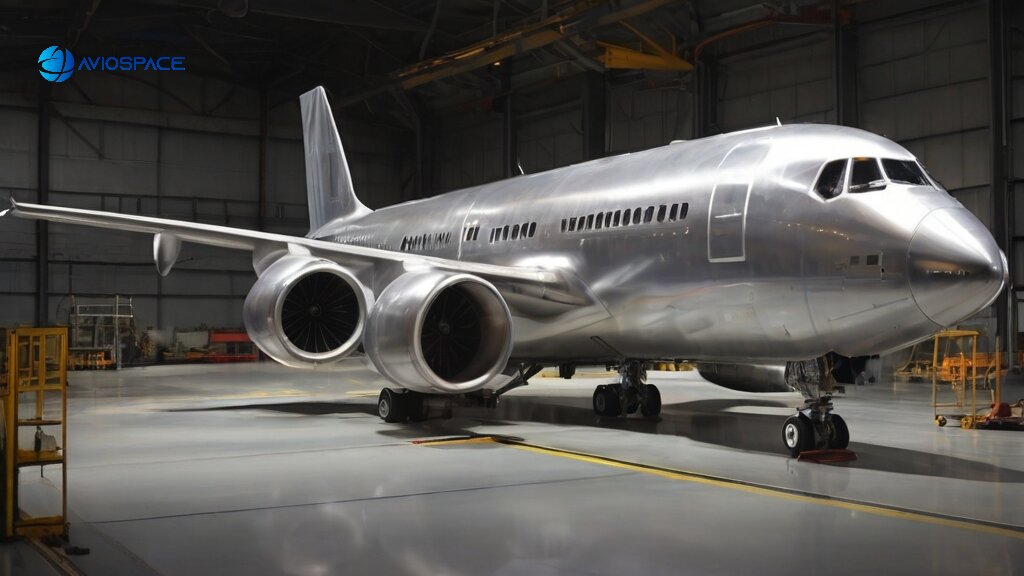
Other Key Areas
The Basement of the orbiter is comprised of tiles made up of Fibrous Refractory Composite Insulation- which is a complex mix of silica fibers, silicon carbide, and aluminoborosilicate fibers. To act as a shield against harsh temperatures, several black tiles were also patched on the body.
With these studies, it is obvious that any aircraft that wants to take to space would demand the addition of several materials that are quite different from the ones used in a normal supersonic aircraft. These added materials would increase the weight of the aircraft and inversely affect its lift generation. Such a tweak in one such factor would dictate trade-offs for other factors, thus creating an imbalance in the manufacturing process.
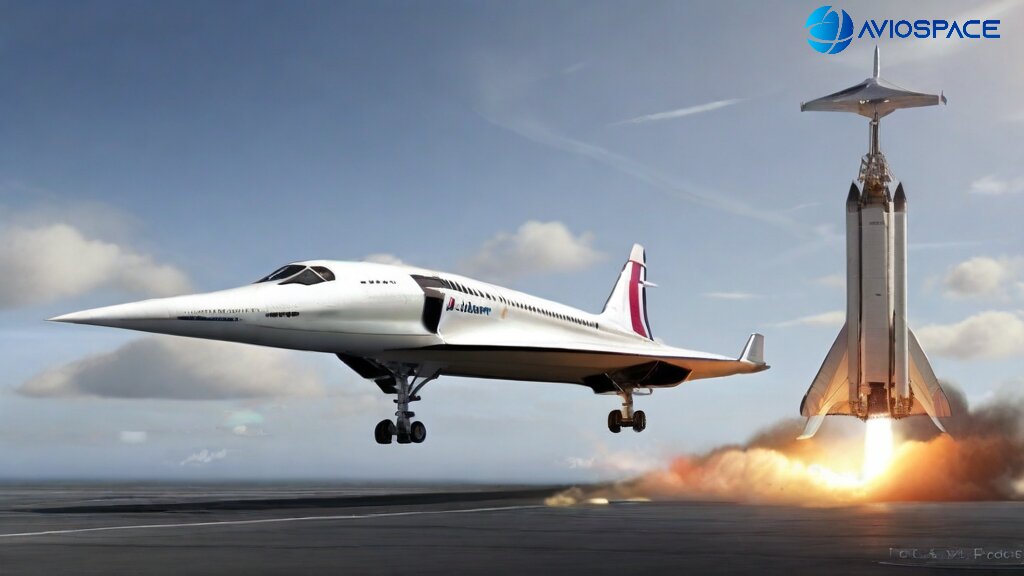
Propulsion Limit
Let’s devise an analytical approach to studying the thrust and engine power that is required for any supersonic aircraft to reach space. The propulsion limit for the journey to space for supersonic aircraft is better explained by the study of two popular missions, i.e., Concorde’s Four Olympus 593 MK.610 Engines and Black Brant XII Launch Vehicle– a sounding rocket developed for suborbital research activities.
It is observed that the thrust produced at the third stage in the sounding rocket is almost 1.5 times that produced by Concorde’s engine in a supersonic cruise at 60,000 feet. The fourth stage, i.e., the rocket’s final stage, generated an average thrust of around 10,592 lb, pushing the rocket above the Karman line, thus surpassing the atmospheric boundary. The thrust generation in the supersonic aircraft stagnated at such altitude, limiting it from achieving the desired thrust as it was at ground level.
Characteristics | Olympus 593 MK.610 Turbojet Engines | Black Brant XII Sounding Rocket |
Engine type | Air-breathing | Non-air-breathing |
Fuel type | Jet A1 | Solid and liquid propellant |
Thrust | 38050 lbs (at take off per engine) | ~ 116,001 lbf (1st stage) |
Thrust | 10000 lbs (at Supersonic cruise) | 15596 lb (3rd stage motor) |
Average Fuel Consumption | 1.195 lb/hr.lbf | Burn time 45 sec |
Total Engines/Stages | 4 | 4 |
Table: Propulsion Comparison chart
The chart clearly shows the extraordinary differences in the engine of a normal aircraft compared to the one used in a spacecraft. We might be able to launch a supersonic aircraft like MiG 25 into space by replacing air-breathing engines with rocket engines but we would also have to work on the overall structural changes, possibly at the expense of a complete makeover.
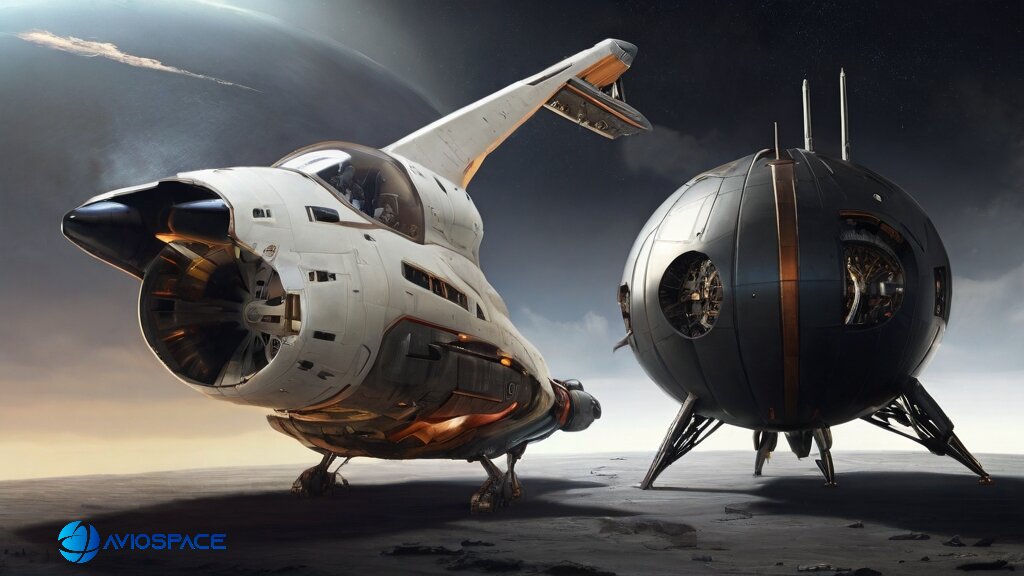
Conclusion
In conclusion, though supersonic aircraft is a marvelous engineering achievement, it is far away from reaching space with its limited speed. The barriers from its structural design don’t allow it to withstand the flaring temperatures demanded by spaceflight. The endeavor requires a different set of challenges, speeds, and technological development essential for space travel and exploration. Therefore, it highlights the necessity of research and development to turn from unattainable to possibilities in years to come.